12 JUNE 2025: THIS WEBSITE WILL BE
REPLACED SOON BY A MORE MODERN ONE
Jobs: NEW PhD positions available (since June
2025)
Position: 1
PhD position (4 years) on 1S-2S spectroscopy of trapped
He+ for fundamental tests, and 1 PhD position for
precision spectroscopy of quantum-degenerate (ultracold)
helium to probe the nuclear size difference between the
alpha and helion particle. Both positions are part of a
new NWO ENW-M2 grant (June 2025)
See the link
below to watch a video explaining
the He+ experiment,
presented by Elmer Gründeman (PhD student), filmed by
Vincent Barbé (Postdoc), and edited by Andrés Matinez
de Velasco (PhD student):
https://youtu.be/bPmsekrWzlw
The first PhD position is available at the
experiment where we want to excite the 1S-2S transition
in a single He+ ion. The aim is to measure the 1S-2S
transition precisely (<10 kHz). He+ is a calculable
system, similar to hydrogen, but with the big advantage
that it can be trapped in a Paul trap, and that Quantum
Electrodynamical (QED) effects are an order of magnitude
bigger than in hydrogen. So precision measurements in
He+ would open up a new testing ground for QED, but also
will enable us to verify the fundamental Rydberg
constant and determine the charge radius of the nucleus:
the alpha particle. This is done by comparing the
results with other determinations, e.g. in atomic
hydrogen, muonic hydrogen, and muonic helium+. The
overall aim is to test the standard model at the
atomic scale, and potentially find new physics.
The second PhD position is available on excitation
of a double-forbidden transition in both 3He and
4He, trapped and cooled to quantum degeneracy (a
degenerate Fermi gas for 3He and a Bose condensate
for 4He). By looking at the spectroscopic difference
(the isotope shift) we can extract the best charge
radius difference of the respective nuclei, the
helion particle and the alpha particle. More details
on this position will follow soon.
The remainder of this page gives more details on the
He+ experiment.
For the He+ experiment there a two main
challenges. The first is the wavelength required to
excite He+ from the 1S state: extreme ultraviolet.
Secondly, the question is how to detect the 1S-2S
transition. Excitation will be performed by a two-photon
transition combining 32 nm and 790 nm. The 32 nm is
produced by high-harmonic generation with ultrafast and
powerful pulses from an amplified frequency comb laser.
Recently we have
been able to excite the He+ 1S-2S transition with 790
nm + 32 nm in ions produced in an atomic beam, which
is a great step forward, and shows that our concept of
excitation works!:
E.L. Gründeman, V. Barbé, Andrés Martinez de Velasco,
C. Roth, M. Collombon, J.J. Krauth, L.S. Dreissen, R.
Taïeb, K.S.E. Eikema
Laser excitation of the 1S-2S transition in
singly-ionized helium
Communications Physics 7, 414 (2024)
https://www.nature.com/articles/s42005-024-01891-4
The next step is a precision measurement, and for
that we will employ Ramsey-comb
spectroscopy, which was developed in our group.
Detection will initially be based on double-ionization.
However, this will destroy the ion, so that a new one
has to be loaded, which takes time. Instead, we want to
develop quantum-logic methods in which we read out the
He+ state by the motion of the Be+ cooling ion that is
co-trapped, see the picture below.
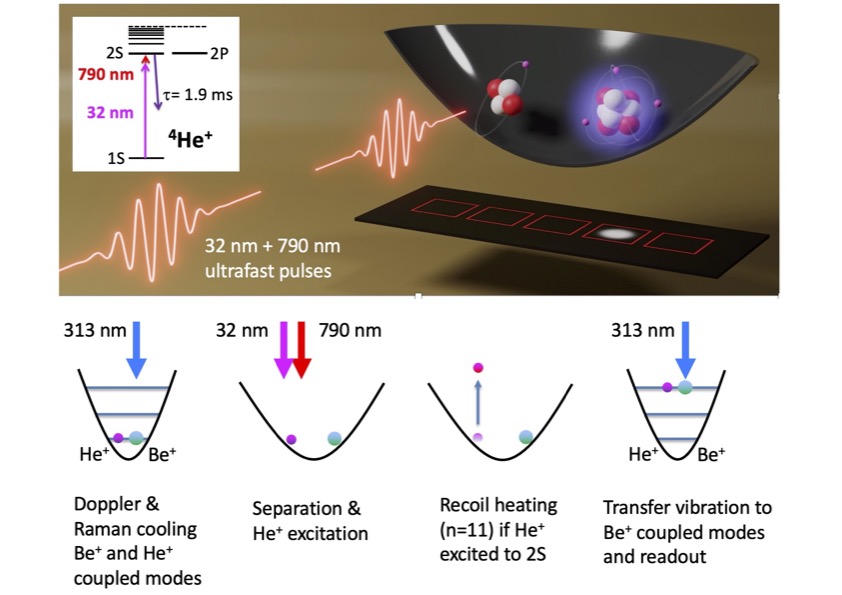
The whole experiment involves many techniques, such as a
frequency comb laser, high-power parametric laser pulse
amplification, an ultra-stable laser, laser cooling at
313 nm, side-band cooling, ultra-high vacuum,
quantum-optics, high-harmonic generation, and so much
more! We have quite unconventional ideas how to realize
the first excitation of He+ from the ground state and
detection of the transition. The target accuracy is 10
kHz or better on the transition.
You can read more about the He+ experiment here.
Below: the High-Harmonic Generation and excitation setup
for He+ spectroscopy (in a configuration for a precursor
experiment with Xe).
The setup has been converted now to excite He+, and are
currently working on driving the 1S-2S in He+ produced
by XUV ionization of He in a beam.

Applicants for PhD position 1 (He+) or PhD position 2
(neutral helium):
You should have a MSc or engineering degree in physics
or something comparable, and have affinity with lasers
and electronics. Most important is that you are bright,
enthusiastic, work well in a team, fluent in English,
are eager to learn, and of course that you are enthusiastic
about these intriguing projects!
General
conditions of employment
You can find information about
the side benefits at
www.workingatvu.nl.
The project and positions are based in the LaserLaB Vrije
Universiteit Amsterdam:
http://www.vu.nl/en/index.asp
The Vrije Universiteit
Amsterdam is a leading, innovative and growing university
that is at the heart of society and actively contributes
to new developments in teaching and research. Our
university has ten faculties which span a wide range of
disciplines, as well as several institutes, foundations,
research centers, and support services. Its campus is
located in the fastest-growing economic region in the
Netherlands (the Zuidas district of Amsterdam), and
provides work for over 4,500 staff and scientific
education for more than 23,000 students.
Evaluations take place
continuously, but as long as this message is
visible, the position is still open.
Send your application to prof. dr. Kjeld Eikema,
email: k.s.e.eikema@vu.nl.
Please include a letter of motivation, CV, and 2 names
with email addresses of people as a reference.
|